Case Report | Peer reviewed |
Cite as: Classen DM, Schwartz KJ, Madson D, et al. Microcystin toxicosis in nursery pigs. J Swine Health Prod. 2017;25(4):198–205.
Also available as a PDF.
SummaryThis case report documents a clinical case of blue-green algae toxicosis, caused by microcystin toxins, in 5-week-old pigs. Mortality during the investigation was elevated by approximately 7.5% in three affected groups, with a final mortality of 11.4%, and 50% of the population demonstrating clinical signs of various degrees. Affected pigs grew slowly and had distended abdomens. Histological examination of tissue samples revealed hepatic centrilobular necrosis with chronic-active periacinar individual hepatocyte necrosis and regeneration or centrilobular hepatocyte necrosis with hemorrhage. Additional testing of the feed revealed no toxicity concerns. Algae were present on the surface of a small area of standing water near the pond that had a waterway to the main water supply. There was a small waterway that connected the standing water to the main pond. Water sampled from that small area tested positive for microcystin. On the basis of these findings, it was determined that the toxicity was caused by algae growth in that area. The affected area was removed to prevent further exposure, and no clinical signs have been present since the standing water area was drained. To the knowledge of the authors, this report describes the first documented case of microcystin toxicosis in nursery pigs. | ResumenEste reporte documenta un caso clínico de intoxicación por algas azul verdosas, causada por las toxinas de microcistinas, en cerdos de 5 semanas de edad. Durante la investigación, la mortalidad se elevó aproximadamente un 7.5% en tres grupos afectados, con una mortalidad final de 11.4%; 50% de la población mostró signos clínicos de varios grados. Los cerdos afectados crecieron lentamente y presentaron abdomen distendido. El examen histológico de muestras de tejido reveló necrosis centrolobulillar hepática con necrosis hepática individual periacinar crónica activa y regeneración o necrosis hepatocítica centrolobulillar con hemorragia. Las pruebas adicionales del alimento no revelaron problemas importantes de intoxicación. Había algas en la superficie de un área pequeña de agua estancada cerca del estanque que tenía un canal al suministro principal de agua. Había un pequeño canal que conectaba el agua estancada con el estanque principal. La muestra del agua de esa pequeña área resultó positiva a la microcistina. En base a estos hallazgos, se determinó que la toxicidad fue causada por el crecimiento de algas en esa área. Se eliminó el área afectada para prevenir mayor exposición, no se han presentado signos clínicos desde que se drenó el área del agua estancada. De acuerdo a lo que los autores, este reporte describe el primer caso documentado de intoxicación por microcistina en lechones de destete. | ResuméLe présent rapport documente un cas clinique de toxicose à la microcystine causé par des cyanobactéries (algues bleu-vert) chez des porcelets âgés de 5 semaines. Le taux de mortalité durant cette enquête était augmenté d’environ 7,5% dans les trois groupes affectés, avec un taux de mortalité final de 11,4%, et 50% de la population montrant des signes cliniques à différents degrés. Les porcs affectés avaient une croissance ralentie et des abdomens distendus. Un examen histologique d’échantillons de tissu a révélé une nécrose hépatique centrilobulaire avec une nécrose chronique-active des hépatocytes péri-acinaires individuels et régénération ou nécrose des hépatocytes centrilobulaires avec hémorragie. Des tests supplémentaires effectués sur l’aliment n’ont pas révélé d’évidence de toxicité. Des algues étaient présentes à la surface d’une petite zone d’eau stagnante proche de l’étang qui avait un canal jusqu’à la source principale d’approvisionnement en eau. Il y avait un petit canal qui connectait l’eau stagnante avec l’étang principal. De l’eau prélevée de la petite zone était positive pour la présence de microcystine. À la lumière de ces résultats, il a été déterminé que la toxicité était causée par la croissance des algues dans cette zone. La zone affectée a été retirée afin de prévenir une exposition future, et aucun signe clinique ne fut détecté suite au drainage de l’eau stagnante. À la connaissance des auteurs, le présent rapport décrit pour la première fois une toxicose liée à la microsystine chez des porcelets en pouponnière. |
Keywords: swine, algae, microcystin, nursery pig
Search the AASV web site
for pages with similar keywords.
Received: September 1, 2016
Accepted: December 7, 2016
Microcystins are toxins produced by freshwater cyanobacteria.1 Microcystin-LR is a common microcystin. When in high concentrations (such as algae blooms), ingested microcystin toxins inhibit phosphatases 1 and 2A in the liver, causing hemorrhaging. Microcystin toxins may also be associated with gastroenteritis and renal necrosis.2 Blue-green algae blooms generally occur during summer months and do not always contain deadly toxins. However, certain species of algae do bloom in the winter.3,4
Some animal facilities in the United States utilize surface water for supply to livestock. The water for ponds is supplied from watersheds during rainfall and snow melt. Field tiles often drain into the watershed supplying the pond. As the water from the pond enters the animal facility, it is generally treated for bacterial and viral pathogens with products such as chlorine, chlorine dioxide, hydrogen peroxide, or ozone.5,6 These products are effective against bacteria and viruses and can help reduce algae growth in the water lines inside the facility; however, they are not effective against pre-formed algae toxins that may already be present in the pond water.7,8
Previous studies1,9 have documented the impact of the toxins on liver function; however, little information is currently present describing cases of accidental ingestion in swine.1,9
Case description
Herd description. This facility was under veterinary care and certified by the Pork Quality Assurance (PQA; National Pork Board). The case herd was established in 2013 as a 2500 breed-to-wean facility maintaining PIC 1050 females, with an attached on-site isolation and gilt developer. The herd was stable for Mycoplasma hyopneumoniae and negative for porcine reproductive and respiratory syndrome virus, porcine epidemic diarrhea virus, porcine delta coronavirus, and transmissible gastroenteritis virus infections. Drinking water for livestock was from an adjacent pond (approximately 17,000 m2) that was naturally filled via watershed. The water was treated with hydrogen peroxide in a holding tank prior to use within the sow facility. The isolation rooms were the point of water entry into the facility. Replacement females were delivered to the facility as isolated weaned pigs at approximately 21 days of age. One hundred and thirty newly weaned gilts were delivered every 28 days. Three days prior to weaning, piglets received 1 mL of Ingelvac Circoflex (Boehringer Ingelheim, St Joseph, Missouri) and 1 mL of Enterisol Ileitis (Boehringer Ingelheim). Additional vaccinations were given to control for porcine parvovirus, Leptospira interrogans serovars, erysipelas, and influenza A virus. Upon selection, gilts were entered into the gestation facility of the farm for their first gestation. A local feed mill manufactured the feed for the facility, with feed formulated to meet or exceed National Research Council10 recommendations.
Clinical description. Pigs presented with clinical signs at approximately 5 weeks of age, starting mid-November 2015. It was suspected that sudden death, the initial clinical presentation, was associated with Streptococcus suis septicemia. Initial treatment to mitigate the potential bacterial infection did not appear successful. The attending veterinarian (DMC) observed that when injectable medications were given, the population did not respond to the therapeutics, and daily and weekly mortality numbers appeared to increase after treatment.
In the three consecutive groups of replacement females affected, the first and third groups (birth dates September 16, 2015, and November 11, 2015) prevalence of clinical signs appeared to be higher, and, in addition, in these three groups, nursery mortality was elevated above the average nursery mortality of 2.3% (Figure 1). Slow growth and distended abdomens were initially reported in both replacement groups (Figure 2). Fifty percent of the pigs were clinically affected and 50% of the mortality was listed as sudden death. No cough or respiratory distress was present and no abnormal bowel movements were noted. No pattern of illness was noted by pen or by room. Paracentesis of the abdomen of euthanized pigs resulted in no fluid collected; however, necropsy of pigs that died demonstrated severe ascites consistently present. Replacement females from the same source as this sow farm did not present with similar clinical signs during the same time period; however, the source farm did have downstream reports of S suis meningitis and septicemia. Tissue samples (brain, colon, heart, joint swab, kidney, liver, lung, mesenteric lymph nodes, oral fluids, serum, small intestine, spinal cord, spleen, stomach contents, tonsil, and urine) were submitted to Iowa State University Veterinary Diagnostic Laboratory (ISU VDL; Ames, Iowa) and University of Minnesota Veterinary Diagnostic Laboratory (UMN VDL; St Paul, Minnesota) at various time points during the period when clinical signs were observed. Both diagnostic laboratories conducted bacteriologic and histopathologic testing, liver mineral panels, and molecular diagnostics. In addition, ISU VDL tested water samples for toxins and for nitrates and nitrites.
Figure 1: A clinical case of blue-green algae toxicosis was caused by microcystin toxins in 5-week-old replacement gilts located in the US Midwest during the fall and winter period of 2015-2016. Animals arrived on farm approximately 21 days after their birth dates and remained on the farm during the course of the observation period in the gilt developer unit. Clinical signs noted in the isolation nursery persisted in the gilt development barn in three cohorts of replacement females. Mortality during the investigation was elevated by approximately 7.5% in the three affected groups, with 50% of the population demonstrating clinical signs of various degrees. Affected pigs grew poorly, had distended abdomens, and histologically exhibited hepatic centrilobular necrosis with chronic-active periacinar individual hepatocyte necrosis and regeneration or centrilobular hepatocyte necrosis with hemorrhage. The percent of mortalities each week throughout the observation period is shown, with the dates representing the birth dates of the cohorts. The August 19th birth group is a baseline group to demonstrate average mortality immediately prior to the first group showing signs of microcystin toxicosis. Birth groups that arrived after the three clinically affected birth groups are presented in the graph to further demonstrate the impact of the toxicosis on mortality.
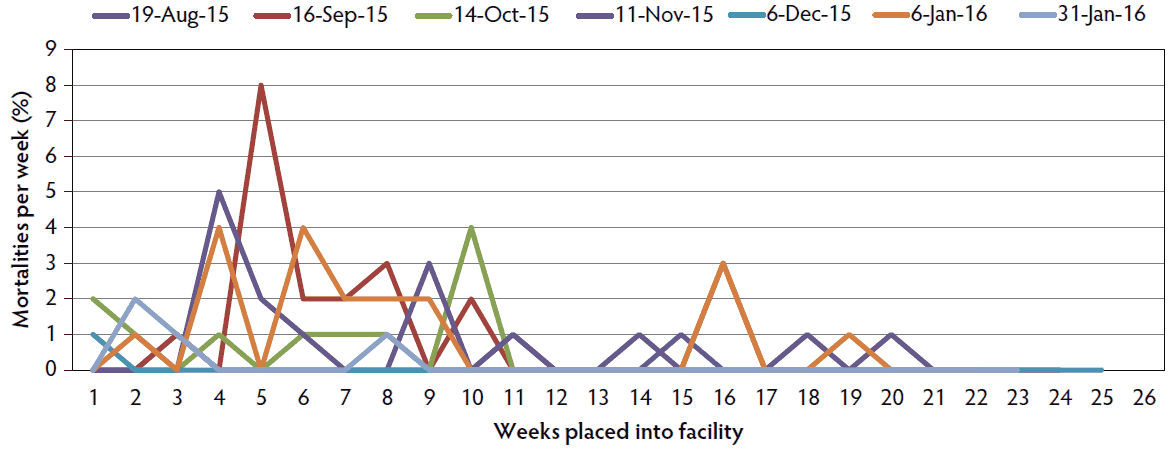
Figure 2: Clinically affected pig, as described in Figure 1, with a distended abdomen (arrow) and poor growth as the initial clinical signs of microcystin toxicosis. Paracentesis of the abdomen of euthanized pigs resulted in no fluid collected; however, necropsy of pigs that died consistently demonstrated severe ascites. Clinical signs appeared at approximately 5 weeks of age, starting mid-November 2015. As described in Figure 1, the prevalence of clinical signs in the three consecutive groups of replacement females affected appeared to be higher in the first and third groups (birth dates September 16, 2015 and November 11, 2015). In all three groups, mortality was elevated above the average nursery mortality of 2.3%.

Laboratory findings. Initial tissue samples submitted in November did not confirm an etiologic diagnosis, hence continued sampling and laboratory submissions occurred as differentials were identified and eliminated from consideration. Samples of feed, serum, tissues, and water were submitted at various times during the period of November 2015 until March 2016 for toxin screening of the feed and water, as well as further pathogen screening and histopathologic and toxicosis evaluations of the tissues. A timeline for sample submission and test results is demonstrated in Figure 3.
Figure 3: Timeline of sample submissions and findings for nursery pigs affected by microcystin toxicity in the case described in Figure 1. CK = creatine kinase; alk phos = alkaline phosphatase; GC/MS = gas chromatography-mass spectrometry.
11/10/2015: Pigs found dead, Streptococcus suis septicemia suspected. Histopathology: no brain lesions; visceral congestion; multifocal centrilobular hepatic necrosis. Chemistry, trace minerals unremarkable. Bacteriology, virology: no signicant ndings. NOTE: Feb 2016: Liver from this submission contained 3 ppb microcystin YR.
12/2/2015: Many pigs now with distended abdomens. Histopathology: severe centrilobular loss of hepatocytes. Bacteriology, virology: no signicant ndings.
12/3/2015: Feed submied for mycotoxin screening. Fumonisin B1 found at 437 ppb in one of two samples.
12/10/2015: Follow-up case. Histopathology: hepatic centrilobular necrosis, extra-medullary hematopoiesis. Bacteriology, virology: no signicant ndings. Clinical pathology: 4/5 aected with elevated troponin vs 0/5 cohorts; high CK and high alk phos in aected pigs. Dierentials for ascites: congestive heart failure, narasin toxicity, hepatopathies, kidney dysfunction, micronutrients, and parasites.
12/31/2015: Liver submied for toxicology screens: GC/MS complete organic toxin screen detected niacinamide and cholesterol (of no diagnostic signicance); trace mineral and toxic mineral analysis within normal limits; 1 ppb of microcystin YR detected in liver. Discussed metals, medications, mycotoxins, chemicals, and plants as causative agents.
12/31/2015: Water sample from isolation, no signicant ndings. Tests: coliforms, pH, trace mineral, GC/MS panel, water quality, microcystins.
1/19/2016: Water samples from pond and holding tank submied with no signicant ndings on pH or microcystins.
1/20/2016: Liver samples submied. Histopathology: consistent with previous cases. Liver from original submission tested for microcystin: positive at 3 ppb for microcystin YR.
2/18/2016: Water sample from isolation room tested specically for microcystins, positive for microcystins LR and RR. Result released 4/15/2016.
3/1/2016: Water and algae sample collected from observation area tested positive for microcystins LR, RR, and YR.
Gross lesions. The predominant changes observed grossly in virtually all pigs were swollen livers with rounded edges and variable fibrous adhesions to the peritoneum (Figure 4). However, most livers had varying degrees of locally extensive hemorrhage and congestion, an accentuated lobular pattern, and often generalized yellowish discoloration consistent with icterus (Figure 5).
Figure 4: Initial necropsy findings of 5-week-old microcystin-affected pigs demonstrated swollen livers with rounded edges and extensive fibrous adhesions to peritoneum (case described in Figure 1).
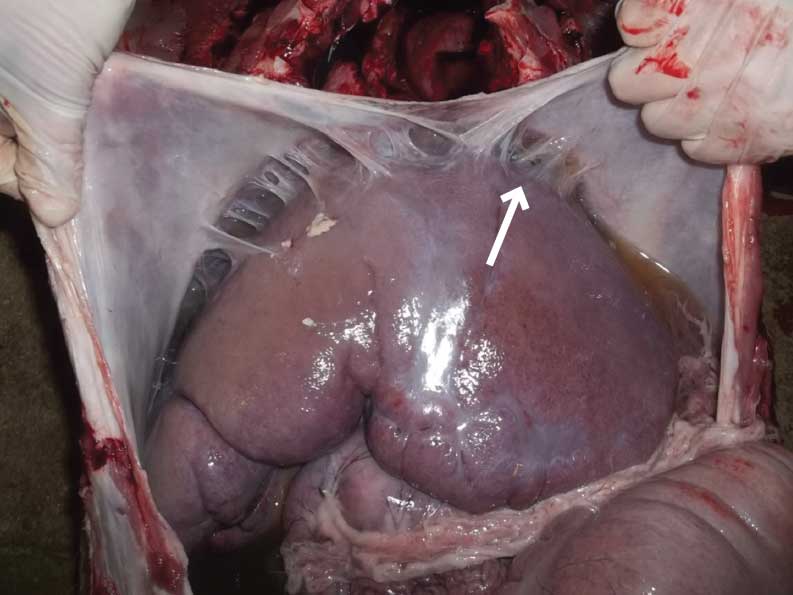
Figure 5: Additional necropsy findings from 5-week-old pigs affected by microcystins demonstrated livers with multifocal centrilobular hepatic necrosis (case described in Figure 1).
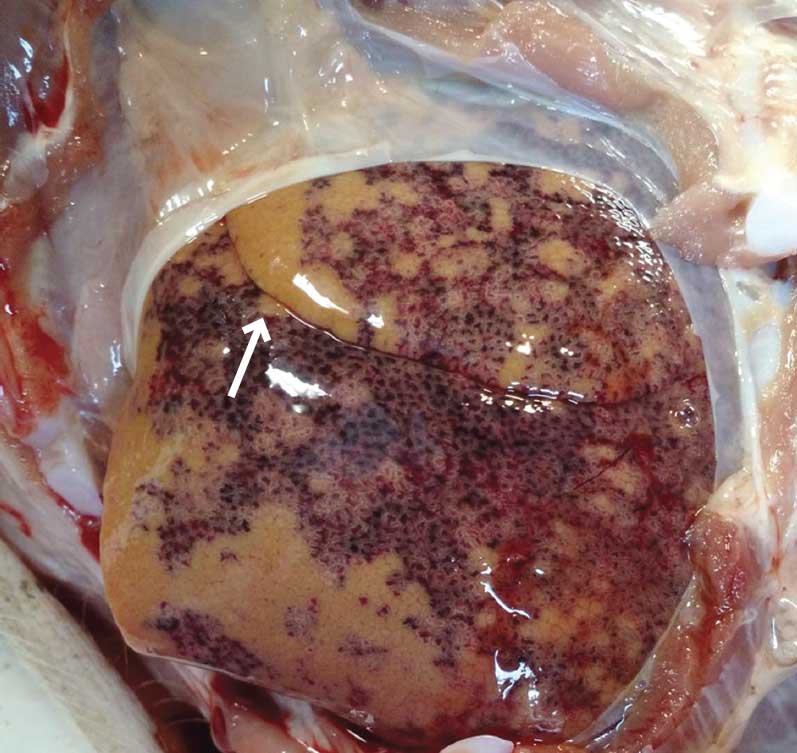
Histopathologic examination. Microscopy of livers in affected pigs presented with massive centrilobular necrosis, with chronic-active periacinar individual hepatocyte necrosis and regeneration, or with severe, diffuse centrilobular hepatocyte necrosis with sinusoidal distention. Lesions appeared subacute in most examined pigs. In addition, centrilobular congestion with loss of hepatocytes and disruption of hepatic cord morphology was a common feature. Hepatocytes in periportal areas were unaffected, and periportal connective tissue, blood vessels, and bile ducts were normal in morphology. Other salient features included scattered foci of extramedullary hematopoiesis and thickening of the liver capsule with fibrosis and fibrin accumulation in some pigs. All other submitted tissues (brain, heart, lung, spleen, spinal cord, and tonsil) were unremarkable. No myocardial lesions were present.
Bacteriologic and virologic testing. Hemophilus parasuis was present in the brain sample collected on November 10, 2015; however, no lesions were detected during histopathologic review of the brain tissue. No significant growth of bacterial pathogens was present in the additional eight sets of samples from subsequent submissions. Neither Mycoplasma hyorhinis nor Hemophilus parasuis was detected by polymerase chain reaction in any samples after November 10, 2015. Likewise, evidence for involvement of porcine circovirus type 2, porcine reproductive and respiratory syndrome virus, and influenza type A virus was consistently lacking by clinical, pathological, and microbiological assessments.
Blood chemistry. Ten blood samples (five from affected pigs and five from clinically normal cohorts) were collected and the serum was submitted December 10, 2015. In all samples, potassium, phosphorus, and alkaline phosphatase (> 130 IU per L) were elevated. Total protein concentrations were low. In three affected pigs, creatine kinase was elevated (> 2500 IU per L), and in four affected pigs, troponin concentrations were elevated (> 0.07 ng per mL). In four affected pigs, aspartate transaminase (AST) concentrations were elevated. Reference ranges provided by the testing diagnostic facility for each of these tests are as follows: potassium, 4 to 7 mEq per L; phosphorus, 4 to 9 mg per dL; alkaline phosphatase, 25 to 130 IU per L; total protein, 7 to 8.9 mg per dL; creatine kinase, 100 to 2500 IU per L; troponin, < 0.07 ng per mL; AST, 10 to 100 IU per L.
Toxin screening. Evaluation of toxic or idiosyncratic hepatic insults in November included screening for drugs, metals, mycotoxins, and organic compounds. Niacinamide and cholesterol were detected in the liver by gas chromatography-mass spectrometry (GC-MS) examination, but were not considered toxic in this case. No unusual organic compounds or concerning concentrations of trace minerals (cadmium, calcium, chromium, cobalt, copper, iron, magnesium, manganese, molybdenum, phosphorus, potassium, selenium, sodium, and zinc) were detected in liver per the reference ranges provided by the testing diagnostic facility. Vitamin E was 3.5 ppm in liver tissue. A second liver mineral screen that included heavy metals such as arsenic, lead, and mercury was conducted on two pigs on December 31, 2015, with similar findings. Additional chemical analysis of liver toxicology screening was performed on January 20, 2016, on tissue collected from the pigs that were submitted on November 10, 2015. One set of the tissues from that date tested positive for 3 ppb of microcystin YR in the liver of an affected pig.
Additional testing. Feed samples from the isolation barn were collected on December 3, 2015, and submitted to North Dakota State University Veterinary Diagnostic Laboratory (Fargo, North Dakota). Testing for a panel of 12 mycotoxins in two feed samples resulted in one non-remarkable finding and one with 437 ppb of fumonisin B1. A single water sample, collected December 31, 2015, from the isolation room, was tested. Water samples were all collected using a clean bottle or container either by holding the container under a water nipple or directly inserting it into the water and holding the container with a clean glove. Water pH conducted by quick test in-house was 7.6. The water was then submitted to the diagnostic facility for additional testing. Tests were conducted by GC-MS for aliphatic hydrocarbons; alkyl benzenes; antioxidants; carbamates; disinfectants; drugs; heavy metals; industrial pollutants; ionophores; natural products; organochlorines; organophosphates; pesticides, plant and fungal toxins; polycyclic aromatics; and vitamins. In addition, the water was tested for coliforms, pH, trace minerals, and microcystins. The findings from that water sample were not significant in that either no chemicals were identified or that the nitrates, pH, sulfates, and total solids all tested below the recommended limits released by the diagnostic facility. Two additional water samples were collected on January 19, 2016, from the pond and the holding tank, with no clinically significant findings associated with pH or microcystins. A single water sample collected from the isolation room on February 18, 2016, held at the ISU VDL, and tested for microcystins in April of 2016, was positive for microcystin LR and RR (3.4 ppb and 2 ppb, respectively). One additional set of three water samples was collected on March 1, 2016. These samples were from an area southeast of the main pond in which there was standing water and algae growing on the surface of the water. Upon further analysis of the area, a small waterway connected the standing water area with the main pond. The three samples collected were just water from that area, and two samples in which the algae was skimmed off the surface to be tested directly. Both of the two algae samples collected from the area tested positive for microcystins LA, LR, RR, and YR (range of 3 to 33.7 ppb LA, 4.3 to 9.9 ppb of LR, 5.4 to 14 ppb of RR, and 4.5 to 4.6 ppb of YR). The sample collected from that standing water area was positive for microcystins LR and RR (1 ppb and 1.6 ppb, respectively).
Additional investigation and outcome. Examination of aquifer and water supply in January 2016 did not reveal algae blooms or discoloration of the main water supply. Snow cover was present during the time of the observations. Additional investigation of the water supply on February 22, 2016, led the attending veterinarian (DMC) to an area of standing water (506 m2) nearby in a wooded area next to the main water supply (Figure 6A). Algae growth was present in this area, and after further evaluation (Figure 6B), it was determined that a small waterway from this area entered the facility’s pond near the point of the intake pump (Figure 6C). Due to the presence of algae during the winter season, DMC documented environmental temperatures (Figure 7) to determine if the temperatures were high enough to support algae growth in the winter. Furthermore, the standing water was removed by reshaping the ground topography to allow for rainfall to flow into the main pond instead of sitting on flat ground. Clinical signs have not returned.
Figure 6: Water supply to the facility in the case described in Figure 1, with the area of observation highlighted in white. This area was separated from the main water supply with a small connecting stream (Panel A; arrow) shown in an image captured February 22, 2016. Closer review showed areas with algae present (Panel B; arrow). The small connecting stream is shown where it entered the main water supply for the facility (Panel C; arrow).
Figure 7: Ambient temperature from November 2015 until March 1, 2016, where the swine facility associated with the case described in Figure 1 was located.

During this period of investigation (November 10, 2015 through March 1, 2016), pigs with severe clinical signs were humanely euthanized. All other animals remained at the facility under observation. As of July 11, 2016, mortality was elevated in all three affected groups identified for birth weeks September 16, 2015, through November 11, 2015. Mortality rates were 15.6% for birth week September 16, 2015; 7.9% for birth week October 14, 2015; and 10.8% for birth week November 11, 2015, compared to the average mortality rate (2.3%) before and after these groups. No new groups have been affected.
Discussion
On the basis of clinical signs and absence of pathogenic bacteria or viruses, despite repeated testing, the authors sequentially investigated potential causes of liver toxicosis other than microcystins. Water was identified as a suspect for carrying a toxin; however, initial findings were unsupported for the water to contain a causative agent. Feed was also considered a source for potential toxins. Feed samples were collected and had a moderate concentration of fumonisin B1, but not at a concentration sufficient to create liver failure.11
The WHO sets a microcystin LR safe allowable limit at 1.045 μg per L (1.045 ppb) for humans.12 Mouse models demonstrate that this concentration results in no long-term consequences when provided for 28 weeks.13 However, animal studies demonstrate that higher concentrations than the allowable limit for long periods of time result in liver injury.14 Acute exposure has been documented and demonstrates the impact of microcystins on liver failure and mortality.1,9 While the duration or dosage of exposure that occurred are unknown in this case, the documentation of microcystin LR concentrations above the human safe allowable limits indicates that liver damage would likely occur.
In this case, the attending veterinarian (DMC) observed that during periods when there were clinical signs in the herd, injectable medications did not reduce the severity of the illness, and mortality appeared to increase after treatment. Upon review of published literature from other species, it was learned that medications that create drug-induced hepatotoxicity should be avoided during periods of liver damage such as cirrhosis.15 Microcystin toxicity results in reduced hepatic perfusion and leads to hepatic failure.16 Drug metabolism is dependent upon both hepatic blood flow and liver enzyme function.17 Furthermore, a single exposure to certain microcystins, such as microcystin LR, can also cause a change in sodium transport in the kidney and result in renal damage.18 Enrofloxacin was used as part of the therapy program in this case; however, past hepatic and renal failure models have demonstrated that enrofloxacin will not cause additional hepatic impairment, but rather, renal impairement.19 Although the primary focus of the case was the liver, because of the distention of the abdomen and coloration of the liver, blood chemistry indicated some kidney damage was present, but no direct link could be made to the use of enrofloxacin and increased mortality. However, on the basis of the literature and known effect of microcystin toxicosis, caution should be placed when selecting appropriate medications for use in animals where microcystin toxicosis is suspected, due to the nature of toxicosis associated with the hepatic and renal functions.
Although initial clinical signs presented in the fall of 2015, new groups of animals became clinically ill during the winter of 2015-2016. The water sample collected from the isolation facility in February, which tested positive for microcystin, indicates that algae growth can occur in the winter and microcystin toxicity can be an issue even during periods of snowfall.3 Upon evaluating ambient temperatures for this timeframe, it can be noted that the temperature was variable, with few days in which the temperature remained below freezing. Algae are likely to release toxins as they die off or are broken down by an animal during digestion, and so it is hypothesized that the toxins were released when the temperatures were below freezing. However, the temperatures did not stay low long enough to kill the algae, and therefore a cycle was established in which the algae grew when temperatures were above freezing and then toxins were periodically released into the water when temperatures fell below freezing. This hypothesis is supported by the evidence of the toxins being identified in the water.4 Winds, snowfall, and freezing rain would all contribute to the standing water in this area, which would then be moved into the main water supply. The small waterway that connected this area to the main pond was near the water intake area. Both algae and the toxin could be moved into the main pond to the water intake area quickly, so little dilution of the toxin could occur before it was moved into the facility. Since the isolation facility was the point of entry into the barn for the water supply, these small pigs were likely to receive the highest concentration of microcystin per kg of metabolic body weight, compared to the remainder of the farm population.
Even though the liver tissue tested positive for microcystin YR in November 2015 and the isolation water tested positive for only LR and RR in February 2016, it is believed that the area of standing water was the contributing factor to this case. Algae can produce all three toxins, which was demonstrated by the March 1, 2016 collection, and therefore it is believed that the toxin presenting in the pig was in higher concentration in November than in February, when the water was collected inside the building, and also in the standing water sample on March 1, 2016.4
Implications
• Under the conditions of this case, fluctuating temperatures may contribute to algae dying and releasing toxins during fall and winter months.
• Surface water should be monitored for algae blooms, but any watershed into those ponds should also be evaluated.
• Use of medications that are hepatotoxic or use the renal pathway should be avoided in animals suspected of having microcystin toxicosis.
Conflict of interest
None reported
Disclaimer
Scientific manuscripts published in the Journal of Swine Health and Production are peer reviewed. However, information on medications, feed, and management techniques may be specific to the research or commercial situation presented in the manuscript. It is the responsibility of the reader to use information responsibly and in accordance with the rules and regulations governing research or the practice of veterinary medicine in their country or region.
References
1. Dawson RM. The toxicology of microcystins. Toxicon. 1998;36:953–962.
2. Van der Merwe D, Sebbag L, Nietfeld JC, Aubel MT, Foss A, Carney E. Investigation of a Microcystis aeruginosa cyanobacterial freshwater harmful algal bloom associated with acute mircocystin toxicosis in a dog. J Vet Diagn Invest. 2012;24:679–687.
*3. Wisconsin Department of Natural Resources. Blue green algae. Available at http://dnr.wi.gov/lakes/bluegreenalgae/. Accessed 29 March 2017.
4. Illinois Environmental Protection Agency. Blue-green algae and harmful algal blooms (HABs). 2015. Available at http://www.epa.illinois.gov/topics/water-quality/monitoring/algal-bloom/index. Accessed 29 March 2017.
*5. Patience JF. Water quality issues in pork production. Proc Leman Swine Conf. St Paul, Minnesota. 2011;157–164.
*6. Pitkin A, Stone S, McCoy J. Examining the impact of water line cleaning, disinfection, and pH reduction on nursery pigs. Proc AASV. Denver, Colorado. 2012;287–290.
*7. Stone S, Pitkin A, McCoy J. Water quality and its effect on production. Proc AASV. San Diego, California. 2013;153–156.
*8. Ramirez A. The science of disinfectants. Proc ISU Swine Dis Conf Swine Pract. Ames, Iowa. 2006;88–97.
9. Jochimsen EM, Carmichael WW, Cardo DM, Cookson ST, Holmes CEM, Antunes MB, de Melo Fiho DA, Lyra TM, Barreto VST, Azevedo SMFO, Jarvis WR. Liver failure and death after exposure to microcystins at a hemodialysis center in Brazil. N Engl J Med. 1998;338:873–878. doi:10.1056/NEJM199803263381304.
10. NRC. Nutrient Requirements of Swine. 11th rev ed. National. Academy Press: Washington, DC. 2012.
11. Casteel SW, Turk FR, Cowart RP, Rottinghaus GE. Chronic toxicity of fumonisin in weanling pigs. J Vet Diagn Invest. 1993;5:413–417.
12. Falconer I, Bartram J, Chorus I, Kuiper-Goodman T, Utkilen H, Burch M, Cood G. Safe levels and safe practices. In: WHO. Toxic Cyanobacteria in Water. A Guide to Their Public Health Consequences, Monitoring, and Management. London, England: St Edmundsbury Press; 1999:161–182.
13. Labine M, Minuk G. Long-term, low-dose exposure to microcystin toxin does not increase the risk of liver tumor development or growth in mice. Hepatol Res. 2014;45:683-692. doi:10.1111/hepr.12394.
14. Chorus I, Bartram J. Human health aspects. In: WHO. Toxic Cyanobacteria in Water. A Guide to Their Public Health Consequences, Monitoring, and Management. London, England: St Edmundsbury Press. 1999;125–160.
15. Amarapurkar DN. Prescribing medications in patients with decompensated liver cirrhosis. Int J Hepatol. 2011;1–5. doi:10.4061/2011/519526.
16. Beasley VR, Lovell RA, Holmes KR, Walcott HE, Schaeffer DJ, Hoffmann WE, Carmichael WW. Microcystin-LR decreases hepatic and renal perfusion, and causes circulatory shock, severe hypoglycemia, and terminal hyperkalemia in intravascularly-dosed swine. J Toxicol Eviron Health Part A. 2000;61:281–303.
17. Rodighiero V. Effects of liver disease on pharmacokinetics. An update. Clin Pharmacokinet. 1999;37:399–431. doi:10.2165/00003088-199937050-00004.
18. Lowe J, Souza-Menezes J, Freire DS, Mattos LJ, Castiglione RC, Barbosa CM, Santiago L, Ferrao FM, Cardoso LH, da Silva RT, Vieira-Beiral HJ, Vieyra A, Morales MM, Azevedo SM, Soares RM. Single sublethal dose of microcystin-LR is responsible for different alterations in biochemical, histological and physiological renal parameters. Toxicon. 2012;59:601–609. http://dx.doi.org/10.1016/j.toxicon.2012.02.003.
19. Hwang YH, Kim MS, Song IB, Lim JH, Park BK, Yun HI. Altered pharmacokinetics of enrofloxacin in experimental models of hepatic and renal impairment. Vet Res Commun. 2009;33:481–487. doi:10.1007/s11259-008-9195-y.
*Non-refered references.